
Silicon, a chemical element denoted by the symbol 'Si' and atomic number 14 on the periodic table, is a lustrous, hard, and brittle crystalline solid with a blue-grey metallic lustre. It is a member of group 14 in the periodic table and is a tetravalent metalloid and semiconductor. It is less reactive than its chemical analog carbon, the nonmetal directly above it in the periodic table, but more reactive than germanium, the metalloid directly below it in the table.
Naturally occurring in the Earth's crust, silicon is the second most abundant element after oxygen, making up approximately 27.7% of the Earth's crust by mass. Silicon is rarely found in its pure state and is usually formed in large, rock-like masses. It occurs mainly in the form of oxides and silicates. Some common examples include quartz, agate, flint, jasper, and amethyst. Certain types of stars are also made largely of silicon.
Silicon is notable for its role in technology due to its semiconductor properties. Semiconductor devices, including transistors, solar cells, rectifiers, and microchips, all rely on silicon. Silicon is also an essential ingredient in the production of steel, and in making ceramics and bricks. It's a common misconception that silicon is synonymous with silicone, a class of silicon-oxygen polymers used in various products, including artificial implants and kitchen utensils. However, silicone is a product of silicon and other elements.
From an industrial standpoint, silicon is typically mined from sand, which is virtually pure silicon dioxide. Once the sand is collected, the silicon is extracted through a heating process. This involves heating the sand with carbon in an electric furnace at temperatures around 2200 degrees Celsius. The resulting reaction produces silicon and carbon dioxide. This method of extraction is known as the carbothermic process.
Silicon is unique in that it possesses properties of both metals and non-metals. Similar to metals, silicon is a good conductor of electricity, and, when polished, it can even reflect light, which is why it is often referred to as a metalloid. Similar to non-metals, silicon is not malleable or ductile; it's quite brittle and shatters when subjected to pressure, which is a characteristic of most non-metallic elements.
In the natural world, silicon plays a vital role in the mineral kingdom. It is a fundamental component in the structure of minerals, and virtually all rocks, clays, and soils contain it. As a testament to silicon's ubiquity, even our bodies contain this element, which contributes to the health of our hair, skin, and nails.
In the metaphysical world, pure silicon crystals are used for their ability to amplify energy and for their beneficial effects on communication skills. As silicon is a natural amplifier, it can be used to enhance the healing properties of other crystals and to strengthen the connection between the physical and mystical realms.
To summarize, silicon is a versatile and abundant element that is integral to both the natural and technological world. Its unique properties make it an indispensable resource in various industries and its potential in the realm of metaphysics and crystal healing is just beginning to be explored. Silicon, in its many forms, continues to play a pivotal role in shaping the world as we know it.
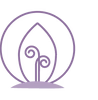
Silicon, the second most abundant element on the Earth's crust, holds a pivotal place in the story of our planet and the universe. An essential building block of life, silicon’s journey begins from the heart of dying stars, and it continues to shape the world around us, including its vast oceans and towering mountains.
To trace the origins of silicon, we need to venture into the cosmos, specifically to the lifecycle of stars. Silicon, like other heavier elements, is born out of nuclear fusion within the fiery cores of massive stars. Stars, primarily composed of hydrogen, function as nuclear reactors. The enormous pressures and temperatures within their cores facilitate the fusion of hydrogen atoms to form helium. This process releases a vast amount of energy, the radiation we perceive as the star's light.
As the star exhausts its hydrogen supply, helium atoms begin to fuse, forming heavier elements such as carbon and oxygen. The fusion process continues, creating even heavier elements, including neon, magnesium, and eventually silicon. This sequence of events is known as stellar nucleosynthesis.
When a massive star has fused most of its atoms into silicon, a significant transformation looms. The fusion of silicon atoms to produce iron signals the star's impending doom. Iron cannot release energy through fusion; hence the star can no longer sustain its nuclear reactions, and the balance between gravity pulling inward and the fusion energy pushing outward collapses. The result is a catastrophic supernova explosion. This explosion disperses silicon and other elements throughout the cosmos, ultimately providing the raw materials for the formation of new stars, planets, and life.
Fast forward to Earth's formation around 4.5 billion years ago. The newborn planet was a molten mass where heavier elements sank toward the center, and lighter ones floated to the surface. Silicon, being lighter than iron but heavier than oxygen, predominantly found its place in the Earth's crust.
Silicon rarely exists in a pure elemental state in the Earth's crust. Instead, it is typically found as silicon dioxide (SiO2), commonly known as silica, and as silicates, the silicon-oxygen compounds that include other elements such as aluminium, magnesium, and iron. Silica and silicates form a variety of minerals, including quartz, feldspar, and mica, which make up most of the Earth's continental crust.
The formation of silicon-based minerals is a complex process involving many factors, including temperature, pressure, and the presence of other elements. Silicon atoms link with oxygen in a tetrahedral arrangement, forming the building blocks of these minerals. Over millions of years, these structures are exposed to heat, pressure, and chemical changes, leading to the formation of various types of rock, including igneous, metamorphic, and sedimentary rocks.
Silicon's journey from the heart of a star to its place in the Earth's crust is a testament to the interconnectedness of the cosmos and our planet. Today, silicon continues to shape our world, not just as a component of rocks, sand, and clay, but also as an essential material in technology, forming the backbone of microchips and solar cells. As we learn more about this remarkable element, we gain a deeper understanding of the universe and our place within it.

Discovery and Extraction of Silicon
Silicon, an element known for its crystalline properties and its essential role in modern technology, accounts for more than a quarter of the Earth's crust by weight. This semi-metallic element, however, doesn't exist independently in nature but is found in various combined states.
Silicon was first isolated in a relatively pure form in 1823 by the Swedish chemist Jöns Jakob Berzelius. However, for thousands of years prior, civilizations were using silicon dioxide, or silica, in the form of quartz and other minerals, without knowing the precise composition of what they were using.
Understanding how silicon is found requires delving into the Earth's crust, where silicon is a major component. It's predominantly found in the form of oxides and silicates. Silicon dioxide is found as quartz, amorphous silica, and even in the cells of diatoms, a type of microscopic algae. The silicates, a vast family of minerals, are more complex and include substances like feldspar, micas, and zeolites, and they contribute to the formation of rocks such as granite, sandstone, and basalt.
One of the most significant sources of silicon is sand, which is primarily composed of silica or silicon dioxide. Mining sand from beaches or riverbeds is a common way to obtain silicon. To extract the silicon, the sand is first purified to remove impurities. This step usually involves washing the sand with water and other chemicals.
After purification, the sand (silicon dioxide) needs to undergo a reduction process. The traditional method for extracting silicon is through a process known as carbothermic reduction. In this process, the purified sand is heated to extremely high temperatures (over 1900 degrees Celsius) in the presence of carbon, typically using charcoal or coke as a source of carbon. The reaction between silicon dioxide and carbon yields liquid silicon and carbon dioxide gas.
This extracted silicon, known as metallurgical-grade silicon (MG-Si), contains impurities and is about 98% to 99% pure. This level of purity is sufficient for many industrial uses, such as in the production of silicates for cement or in the making of silicones, a family of synthetic polymers.
However, for the silicon to be used in electronic applications or solar cells, it needs to be further purified to semiconductor-grade silicon. This is achieved through a process known as the Siemens process, where the MG-Si is reacted with hydrogen chloride to produce trichlorosilane. This substance is then subjected to chemical vapor deposition, resulting in the formation of an ultra-pure form of silicon.
It's worth mentioning that while silicon is abundantly available, the processes of extraction and purification are energy-intensive, and care needs to be taken to mitigate the environmental impacts associated with these processes.
The discovery and extraction of silicon have been instrumental in shaping the modern world. From the silicon chips at the heart of our computers and smartphones to the silicon cells in solar panels, this element continues to transform our society. As we continue to find new applications for silicon and better ways to extract it, our understanding of this remarkable element continues to evolve.

Silicon, despite being a crucial component of the Earth's crust and a critical element in modern technology, has a history that is less well-known than some of the other elements. Nevertheless, its journey from the Earth's mantle to the core of technological devices is nothing short of fascinating.
The story of silicon begins deep within the Earth's mantle, where it forms in large rock-like masses. Silicon does not exist naturally in its elemental form due to its high reactivity; instead, it is typically found as a component of silicate minerals. These minerals, including quartz, mica, feldspar, and clay, are abundant in the Earth's crust and were known to our ancestors, although they didn't understand their silicon content.
Silicon's journey to recognition began in 1787, when French chemist Antoine Lavoisier suggested that a new element might be part of the mineral quartz. However, it wasn't until 1824 that the element was finally isolated. Jöns Jakob Berzelius, a Swedish chemist, heated chips of potassium metal with silica inside a glass tube. The potassium reacted with the silica to form potassium silicate, which was then washed away with water to leave behind a residue of silicon. This was the first time that silicon was prepared in its elemental form, and Berzelius is generally credited with its discovery.
In the 19th and early 20th centuries, silicon was primarily used in steel refining and as a component of aluminum-silicon alloys. It was valued for its ability to remove oxygen from metal ores and its hard, brittle properties that contributed to the strength of alloys.
The silicon industry truly took off in the mid-20th century, however, with the advent of the semiconductor industry. During the 1950s and 1960s, scientists learned to grow single-crystal silicon for use in semiconductors, a breakthrough that laid the foundation for modern electronics. Silicon's properties as a semiconductor, particularly its ability to amplify electrical signals and its high resistance to heat, made it the perfect material for these applications.
The use of silicon in computer chips has been a defining feature of the Information Age. Silicon Valley, the southern region of the San Francisco Bay Area in Northern California, was named for the silicon transistor and is now home to many high-tech corporations and thousands of startup companies.
On the metaphysical side, the pure silicon crystal is believed to have been used by ancient civilizations, though no specific records exist. Its mystical uses have been largely developed in recent decades, in alignment with the New Age movement. It is often used for its ability to amplify energy and communication skills.
Today, silicon remains an essential element in both the physical and digital worlds. From its early formation in the Earth's crust to its pivotal role in the development of technology, silicon's journey is a testament to its unique properties and versatility. As we continue to find new applications for this remarkable element, the history of silicon is still being written.

Silicon crystals, despite being a critical element in modern technology, are not traditionally steeped in legends and lore. However, their metaphysical attributes have become an integral part of New Age philosophy, and modern myths have evolved surrounding their perceived power. They have become a symbol of the link between the ancient natural world and our modern technological society.
One such modern myth is the notion of "Programmable Crystals." This concept hinges on the idea that silicon, being a vital component of computer chips, can be imbued or "programmed" with human intentions. According to this belief, holding a silicon crystal and focusing one's intention or desire onto the crystal can "program" it. This is a kind of modern divination, linking ancient ritual with the language of our current digital age.
This notion has grown to suggest that silicon crystals can store, amplify, and transfer energy. Some spiritual practitioners believe that these crystals can be used in healing practices, acting as a tool to direct healing energies into the body. In a broader sense, it's believed that they can help in manifesting desires into reality, a concept similar to the Law of Attraction.
Another legend that has grown around silicon crystals, particularly quartz (composed of silicon dioxide), is the idea of the "Crystal Skulls." These are human skull hardstone carvings often attributed to ancient Mesoamerican cultures. The mythology around these artifacts suggests they could be of ancient or even extraterrestrial origin and hold great power or wisdom. While this myth is compelling and has been popularized by media, no scientific evidence supports these claims, and many of the skulls are recognized as 19th-century fakes. However, this legend exemplifies the allure and mystery that people associate with silicon-based crystals.
Moreover, silicon is central to the mythos of our modern digital world. We find it embedded in every aspect of our lives, from our computers to our cell phones. Silicon Valley, named for the silicon transistor, is a place of legends in its own right. Tales of startups becoming billion-dollar corporations, of inventors becoming modern-day heroes, add to the lore of silicon. In a way, these contemporary legends echo the traditional tales of transformation and alchemy.
On a broader metaphysical level, silicon crystals symbolize our quest for knowledge and communication, a bridge between the earthly and digital realms. Just as these crystals have become an essential component of our technology, they also represent a spiritual tool in our quest for greater understanding and connectivity.
Despite the lack of ancient legends, the allure and mystique of silicon crystals lie in their scientific properties, their versatile uses, and the modern myths and perceptions that we have constructed around them. In essence, silicon crystals encapsulate the interplay between the natural world and human innovation, becoming legends in their own right in our collective consciousness.

Long before the birth of technology as we know it today, when humankind was still in its infancy, the planet was ruled by powerful elemental beings. Each element - earth, water, air, and fire - had a guardian, a titanic being tasked with maintaining the balance of their element in the world. Among them, the earth guardian, Terros, was a colossal figure composed of numerous minerals and rocks, reflecting the richness of the Earth itself.
Deep within the heart of Terros, hidden in his core, was a mysterious crystal unlike any other, an object of unparalleled beauty and remarkable power. This was the Silicon Crystal, the very source of Terros' immense strength. The Silicon Crystal had the unique ability to communicate with all the rocks and minerals of the Earth, allowing Terros to sense every tremor, every shift, every formation, and every erosion that occurred on the planet.
One day, Terros sensed a new energy stirring within the depths of the Earth. The humans, who had been mostly hunters and gatherers until then, had discovered the art of smelting and were starting to use tools made from metal. Intrigued by their ingenuity and potential, Terros decided to bless them with a gift, a piece of his very own heart - the Silicon Crystal.
To prevent it from causing harm if it fell into the wrong hands, Terros transformed the Silicon Crystal into countless tiny grains of sand, spreading them all over the Earth's surface. He then whispered a riddle to the winds, a clue to the humans about the true nature of this gift, "The power to connect all rests in the heart of the humble grain."
Centuries passed, civilizations rose and fell, and the sands of time flowed on, the riddle and the secret of the Silicon Crystal hidden within them. The sands were used in construction, glass-making, and numerous other crafts, but their true potential remained undiscovered.
Then came the Age of Enlightenment. Science and reason became the guiding lights of human progress. People started digging deeper, both into the Earth and into their minds, in search of knowledge and power.
One day, a young alchemist named Berzelius stumbled upon the ancient riddle of Terros. Intrigued, he began a quest to uncover its meaning. He studied the sands, observed their composition, and, after countless experiments, managed to isolate a strange material from the grains – a semi-metallic element he named Silicon.
Berzelius found that Silicon had an extraordinary ability to transmit energy. But the world was not yet ready for such a revelation, and so Silicon, like sand, slipped through the fingers of progress, waiting for its time to come.
It wasn't until the dawn of the 20th century that the power of the Silicon Crystal was truly unleashed. With the invention of the transistor and later the silicon chip, the Digital Age began. Silicon, in its crystalline form, became the heart of this new era, driving technologies that connected people across the globe, just as Terros had once connected with the rocks and minerals of the Earth.
As we moved further into the 21st century, the applications of silicon crystal evolved even further. It was not only a material for electronic devices but also an essential component of solar panels, turning sunlight into electricity and playing a key role in the transition to sustainable energy sources.
And so, the legacy of the Silicon Crystal continues, from the heart of an ancient elemental guardian to the heart of modern technology and sustainable energy solutions. In its journey, the Silicon Crystal has connected not only all the rocks and minerals of the Earth but all of humankind, fulfilling the prophecy of Terros's riddle. From the humble grain of sand to the nucleus of our digital world, the legend of the Silicon Crystal stands as a testament to human curiosity, ingenuity, and the enduring power of the elements.

Silicon crystals, despite their common occurrence in the natural world, hold an underappreciated mystical aura. These tiny powerhouses form the backbone of modern technology, bridging the gap between the physical and digital realms, making them an important symbol for connection, transformation, and progression in metaphysical practices.
One of the key mystical properties attributed to Silicon is its role as an energy enhancer. It is said to absorb, store, release, and regulate energy, much like it does in the world of technology, where it forms the core of transistors and integrated circuits. This property makes Silicon crystals an essential tool in energy healing, where they can amplify the healing intentions and energies of the practitioner and channel them more effectively.
Silicon crystals are also known for their grounding properties. Much like Silicon forms the Earth's crust, connecting every landmass and island, it is believed to aid in establishing a deep connection to Earth. This grounding effect helps balance one's energies and attunes them to the Earth's frequency, facilitating a sense of stability and security.
Moreover, due to their capacity for energy transference and regulation, Silicon crystals are believed to be excellent tools for telepathic communication. They are thought to aid in sending and receiving messages across the astral plane, amplifying one's innate psychic abilities. Their strong communicative properties resonate well with the Throat and Third Eye chakras, making Silicon crystals a valuable asset for anyone seeking to enhance their clairvoyance or clairaudience.
In addition, Silicon is associated with transformation. This property is linked to Silicon's role in modern technology, transforming the way we live, communicate, and perceive the world. On a personal level, Silicon crystals can be used in spiritual practices to inspire personal transformation, stimulate adaptability, and encourage innovation and progress.
One of the more unique mystical properties of Silicon is its connection to the realm of dreams. Believers in crystal healing often use Silicon crystals to enhance their dream recall and understanding. This stone is said to stimulate the subconscious and make one's dreams more vivid, thereby assisting in unraveling the symbolic messages our subconscious mind communicates through our dreams.
Last but not least, Silicon crystals are considered a manifestation tool, owing to their capacity to store and amplify energy. When programmed with a specific intention, Silicon crystals can continuously broadcast that intention, helping to manifest it into reality.
In essence, the mystical properties of Silicon crystals resonate with the energy of advancement and progression that defines the technological age we live in. They ground us, enhance our communication, foster personal transformation, illuminate our dreams, and help bring our intentions to life. As our world becomes more intertwined with technology, the mystique of Silicon crystals offers an intriguing path for bridging our physical existence with the digital cosmos.

In the world of magic and crystal healing, Silicon, particularly in the form of quartz, holds a unique and powerful position. Despite not having ancient tales or traditions surrounding its use, silicon-based crystals have come to play a vital role in contemporary spiritual and metaphysical practices. Here are some suggestions for how you might use silicon crystals in your own magical practices.
Programming and Manifestation
Silicon is a key component in computer technology and, in the metaphysical world, this characteristic has been translated into the concept of 'programming' your crystals. Programming a silicon crystal involves focusing your intentions or desires into the crystal, essentially imbuing it with a specific purpose or goal. This is particularly powerful when done in conjunction with manifestation work.
To program your silicon crystal, sit quietly and hold the crystal in your hand. Clear your mind and focus on your intention or desire. Visualize this intention as energy moving from your mind, down your arm, and into the crystal. Spend several minutes, or even up to an hour, focusing on this process. When finished, your crystal is 'programmed' and can act as a physical reminder and amplifier of your intention.
Healing and Energy Work
Silicon crystals are also believed to have significant healing properties. They are said to aid in mental clarity and focus, making them an excellent tool for meditation and healing practices. They can also help to cleanse and balance the chakras, particularly the Crown chakra, enhancing your spiritual connection.
In energy healing, silicon crystals can be used to direct healing energies into the body. Place the crystal on the area in need of healing, imagining the crystal's energy moving into your body to facilitate the healing process. For a full-body energy cleanse, place several silicon crystals around your body in a grid-like pattern, and meditate, visualizing any negative energy being drawn out and replaced with positive, healing energy.
Enhancing Psychic Abilities
Silicon crystals are also associated with enhancing psychic abilities such as clairvoyance, telepathy, and intuition. Use a silicon crystal during meditation to open and activate the Third Eye chakra, which is believed to be the center of these abilities. Hold the crystal in your hand or place it on your forehead to enhance this process.
Balancing Digital Energies
In our modern digital world, we are continually surrounded by technology. The silicon in our devices holds immense energy, and some people believe that this can affect our own energy fields. Using silicon crystals, particularly clear quartz, can help to balance out these digital energies. Place silicon crystals around your home, particularly near your electronic devices, to help harmonize the energy in your space.
In Ritual and Spellwork
Silicon crystals can also be used in a variety of magical rituals and spells. Incorporate them into rituals for clarity, healing, or spiritual growth. Use them in spells for manifesting goals or desires. They can also be used in divination, either by casting stones or as a focus during tarot readings or other divination practices.
In conclusion, while silicon may not have a long history of magical use, it has a significant role in contemporary crystal magic. As a bridge between the natural world and our modern technological society, silicon crystals offer unique and powerful energies for our magical practices. As with all magical practices, remember that the key is your intention and focus. A crystal is a tool, and its power comes from you.